The Weight and Balance of Air Midwest 5481
Air Midwest, headquartered in Wichita, Kansas, was originally certified as an air carrier by the FAA in 1965. The airline originally consisted of a single Cessna 206, which transported human remains for area mortuaries. In 1968, the company began to provide a passenger service (for live passengers). The Mesa Air Group acquired the company in 1991. As a subsidiary of Mesa, Air Midwest operated 12 Beechcraft 1900D’s.
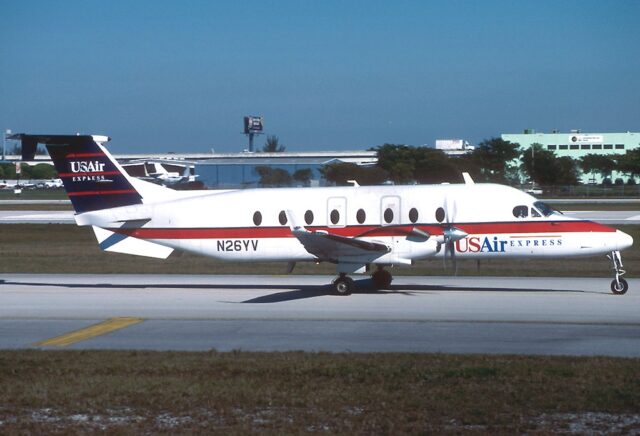
The Beechcraft 1900 is a 19-passenger twin-engine turboprop which is popular with regional airlines. It is very fuel-efficient over short routes and is able to frequent small airfields with short or grass runways. The original design had two passenger boarding doors with built-in steps, one at the front and one at the rear. The next model replaced the aft door with an enlarged cargo door, as a nineteen-seater plane really doesn’t need two points of access. The 1900D expanded the cabin height in order to allow passengers to walk upright. The 1900D also has more powerful engines, modified propellers, winglets and a larger tail. It is the most popular version of the Beechcraft 1900 series.
On the night of the 6th of January 2003, the Beechcraft 1900D registered N233YV was brought in for a maintenance check. It was returned to service on the morning of the 7th of January.
That day, the aircraft flew six flight legs, with the final flight from Huntington, West Virginia to Charlotte-Douglas International Airport (CLT) in Charlotte, North Carolina. The captain was the Pilot Flying on that last leg and, after the flight, the first officer, as Pilot Monitoring, commented that, “everything was normal” and “it was a good flying airplane.” Nothing seemed amiss.
A different crew then used the aircraft for a flight to Lynchburg, Virginia. That crew returned the aircraft to Charlotte-Douglas International Airport the following morning. They did not report any issues with the aircraft.
That morning, the 8th of January, the original flight crew was scheduled to fly two legs: from Charlotte to Greer, South Carolina and then from Greer to Raleigh-Durham, North Carolina. From there, they would travel as passengers back to Charlotte.
The captain arrived at the gate at 07:45 and the first officer arrived at 08:00. All times are in eastern standard time, the local time zone for Charlotte.
The first officer did a walk-around inspection of the aircraft. He did not report anything unusual. There were 19 passengers for the morning flight to Greer. The dispatch release for Air Midwest flight 5481 showed that a maximum of 23 bags were allowed on the flight.
One of the two ramp agents working the flight said afterwards that there were 23 checked bags and 8 bags taken onto the aircraft as carry-on. The forward cargo compartment was about 98% full by volume. The ramp agent mentioned to the captain that two of the bags were heavy although they were not marked as such. She responded that it didn’t matter, as there was a child passenger on the manifest whose weight had been included using the average adult weight, so it would balance out.
The flight crew entered the cockpit and completed the pre-flight paperwork, including the required weight and balance calculations.
Proper weight and balance control is critical to the safe operation of any aircraft. The three elements of weight and balance are:
- the weight of the aircraft
- correct weight and balance records
- proper loading of the aircraft
Any one of these elements is meaningless if all three are not correct.
The pilot in command is responsible for the aircraft loading, crew, passengers, baggage/cargo and fuel, to ensure that the centre of gravity is within limits and the maximum allowable weight is not exceeded.
Generally speaking, people are not individually weighed and it is common to use average figures for passengers and their bags. In 2003, Air Midwest and many other airlines used average figures as released by the FAA some years earlier: 170 lbs for an adult, 80 lbs for a child up to 12, and 30 lbs for a checked-in suitcase. For reference, that’s 77 kilos for an adult, 36 kilos for a child and 13.5 kilos for a checked-in suitcase. From here on out, I will stick to pounds for consistency.
The Beechcraft 1900D, registration N233YV, weighed 10,293 pounds. The load manifest form for flight 5481 lists the aircraft’s empty weight as 10,673 pounds: the aircraft weight, 170 pounds for each pilot and 20 pounds per bag (one per pilot).
That day they had 19 passengers and Air Midwest used an average weight of 175 pounds per adult and 80 pounds per child. The passengers were all counted as adults, making for a total of 3,325 pounds.
The next step was to add the cargo weight. The weight in the coat closet was estimated as 10 pounds. The luggage was estimated using an average weight of 25 pounds. The load manifest form listed the forward (AFT1) cargo compartment estimated at 775 pounds and the aft (AFT2) cargo compartment at 45 pounds.
Although aviation fuel is dispensed in gallons in the U.S., it is converted to and referred to in pounds once in the aircraft’s fuel tanks, specifically because the weight of the fuel is required for the weight and balance checks.
The aircraft was loaded with 2,420 pounds of fuel. Air Midwest operations estimate 110 pounds of fuel are burned taxiing to the runway. Thus, 2,310 pounds of fuel would be the correct amount to add to the load manifest; the figure was entered as 2,200 pounds, 110 pounds short, likely a case of subtracting the fuel required for taxi twice. The form also had an addition error of ten pounds.
The final figure on manifest showed that the take-off weight of N233YV for flight 4581 was 17,018 pounds.
The Beechcraft 1900D maximum gross take-off weight is 17,120 pounds, which means that it appeared to be within limits.
The crew performed pre-taxi checks, including a control check of the elevators, which are hinged to the back of the horizontal stabiliser. The elevator moves up and down to control the aircraft’s pitch, climb and angle of attack. The control check is performed by moving the control column from the full forward position to the full aft position. The range of motion on the control column was normal; the elevators were clear and correct. The flight crew did not notice any issue during any of the pre-flight checks.
The captain was the Pilot Flying and the first officer was the Pilot Monitoring.
The first officer contacted the air traffic control tower to say that flight 5481 was ready to taxi. The ground controller instructed them to taxi to runway 18R. About ten minutes later, the tower controller cleared flight 5481 for take-off, with the instruction to turn right for a heading of 230° after take-off.
The captain asked the first officer to set the take-off power and the first officer responded that the power had been set. They were ready to go.
The rotation speed (VR) of the Beechcraft 1900D, the speed at which the aircraft can safely lift off the runway, is 105 knots. At 08:46:48, the aircraft’s airspeed was above 102 knots and the elevator position was 7° nose down. About three seconds later, the elevator position was 1° nose down and the pitch attitude began to increase as the aircraft took off. At 08:46:53, the pitch trim started moving nose down. About three seconds later, the captain called for the landing gear to be retracted. The elevator position returned to 7° nose down and the cockpit voice recorder recorded the sound of the landing gear retracting.
In the cockpit, the voice recorder captured the first officer saying “Wuh?” The Captain said “Oh,” followed by “Help me!”
The aircraft was 90 feet above the ground and the nose was dangerously high. The pitch attitude was 20° nose up, the aircraft climbing a steeper ascent than it could possibly maintain.
The captain asked, “You got it?” The flight data recorder shows that both flight crew were forcefully pushing the nose down. During the next eight seconds, they continued to try to force the aircraft’s nose down.
The stall warning horn sounded. Twenty seconds after take off, the pitch altitude was 54° nose up. The captain contacted ATC with a mayday call. “We have an emergency for Air Midwest 5481.”
The stall warning horn stopped. The aircraft pitch attitude decreased through neutral (0°) and the elevator began to move nose up.
The aircrew almost regained control of the aircraft but not for long. At 1,150 feet above the ground, the aircraft began to roll to the left. The flight data recorder recorded a left roll of 127° and the airspeed as low as 31 knots. A second later, the pitch attitude was 42° nose down.
“Pull the power back,” said the captain. The elevator position reached full nose up. The stall warning horn sounded again. The flight crew managed to get the nose down and then stabilised the aircraft roll but the pitch attitude increased again. Then, the aircraft rolled to the right with a vertical acceleration of 1.9Gs. The transcript of the voice recorder show just how impossible it was to do anything: the captain called out “Oh my god, aah!” as her first officer said, “Oh god, [expletive]”.
The cockpit voice recording ended two seconds later and the emergency locator transmitter signal began transmitting as the aircraft crashed into a US airways maintenance hangar.
The last recorded pitch attitude was 47° nose down, roll attitude 66° to the right, pitch control position 19.2° nose up.
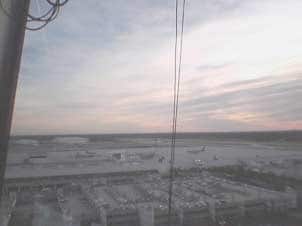
There were seven air traffic controllers in the air traffic control tower when the accident happened, three of whom had dealt with flight 5481 that day: the clearance delivery controller, the ground control west controller, and the local control west controller.
The local control west controller was the first to notice that Flight 5481 was in difficulties. He said that the aircraft lifted up about 3,200 feet down the runway and started a normal climb that kept getting steeper. He called out, “That guy’s gonna stall.”
The ground control west controller looked to see the aircraft in a steep climb from about 200 feet. Instead of turning to the right, as expected as a part of its departure, the Beechcraft 1900D continued to pitch higher. By the time the flight was at 800 to 1,000 feet it was almost vertical.
At about 1,000 feet, the aircraft fell to the left and then descended, nose down, as it picked up speed.
Someone yelled, “Crash phone!”
The ground control east controller picked up the phone while the aircraft was still in the air. She saw flight 5481 level off at about the height of the US Airways hangar, which was likely when the captain briefly recovered control. But then the nose dropped again.
The local control west controller said he saw the aircraft flip upside down. As the aircraft impacted the hangar, the crash phone was answered.
“An emergency…landing right now at the US Air maintenance ramp. Right at the corner of the building — there’s a fire.”
The aircraft crashed into the hangar and stopped, having travelled about 7,600 feet (2,316 metres) from the runway threshold.
The emergency response was immediate but it was no good. The accident was not survivable.
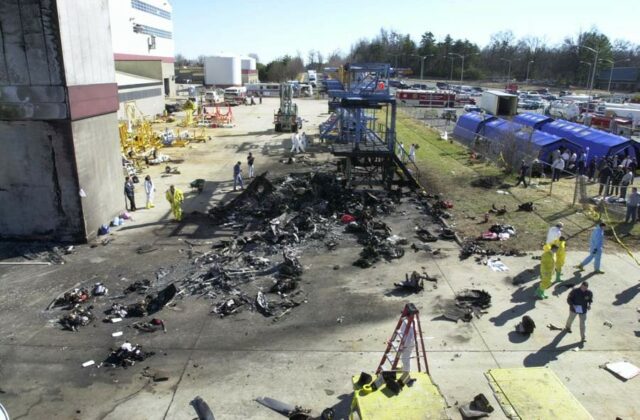
The main wreckage was about 30 feet (9 metres) west of the southwest corner of the US Airways maintenance hangar.
The primary impact was the northwest front side of the hangar wall. The wall was constructed of reinforced cinder block with exterior metal flashing that started about 20 feet (6 metres) above the ground to the roof. The wall had a large hole and was cracked both vertically and horizontally. Several pieces of the wall material were found mixed with the aircraft wreckage. The nose of the aircraft was crushed. The left engine was lying upright and displaced about 45° to the left of the airframe centreline.
The cause of death for all on board was declared as “multiple blunt force injuries due to airplane crash,” which means that they were killed in the impact and dead before the fire started.
One of the oddities of the wreckage was that the captain’s body was found about four feet away from the cockpit wreckage. The captain’s seatbelt buckle was found unlatched in the cockpit wreckage although the first officer’s was still attached. However, the medical examiner was clear that she had also died in the impact.
Investigators recreated the scenario with a test subject around the captain’s height and weight in the seat, with the seat full forward and full up. If he or she pulled the control wheel fully aft and rotated it clockwise, they found that the control wheel consistently knocked the seatbelt buckle and released it. Thus, as she was struggling to recover the aircraft from its crazy pitch and roll, the seatbelt buckle came undone and she was then knocked out of the cockpit in the crash.
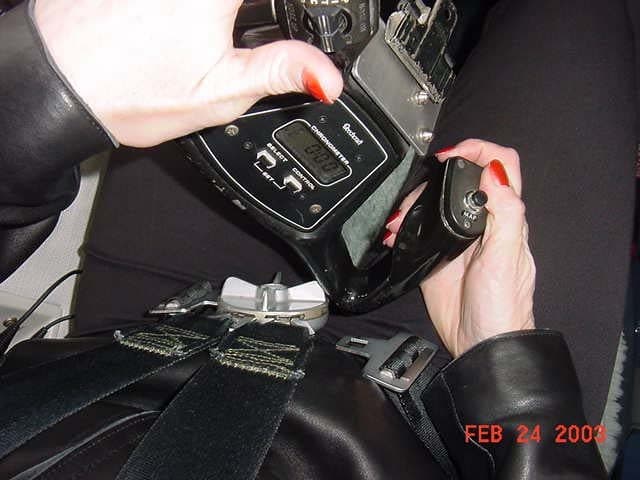
The accident had all the hallmarks of an aircraft outside of its weight and balance envelope. An aircraft has a maximum weight, which is based on the amount of lift that the wings can provide and may also be limited by the structural strength of the fuselage. The dynamic loads on an aircraft are increased by flight manoeuvres.
The wings of a 3,000-pound aircraft need to support 3,000 pounds in level flight, but when the aircraft is turned smoothly and sharply using a bank angle of 60°, the dynamic load requires the wings to support twice this weight, in our example, 6,000 pounds. The structure must be strong enough to support a load factor of 3.8 times its weight in order to ensure the aircraft is not at risk during uncoordinated manoeuvres or turbulence.
The ideal location for the centre of gravity, along with a maximum deviation, is determined by the designers. This can be thought of as a see-saw, where the see-saw is balanced when the weight on one end multiplied by its distance from the middle is equal to the weight on the other end multiplied by its distance from the middle—the bigger kid needs to sit nearer to the fulcrum for it to balance. In the same way, you can modify the centre of gravity of an aircraft by moving passengers to other seats or shifting cargo to another compartment.
On an aircraft, the fulcrum is at the wings but we need to be more specific than that, taking into account the shape of the wing. The Mean Aerodynamic Chord (MAC) is the front-to-back line on the wing where the aerodynamic force and position is the geographic centre of the area of the wing. This means that the centre of gravity of the aircraft needs to be located along that chord, usually somewhat towards the front.
As a result, the centre of gravity position is shown as a percentage MAC. The Beech 1900D aft centre of gravity limit is 40% MAC.
The FAA explain the MAC in the Aircraft Weight and Balance Handbook:
The MAC is the chord of an imaginary airfoil that has all of the aerodynamic characteristics of the actual airfoil. The chord is drawn through the geographic center of the plan area of the wing. The location of the CG with respect to the MAC is important because it predicts the handling characteristics of the aircraft.
The Air Midwest centre of gravity take-off limits range for the Beech 1900D aircraft with a take-off weight of 17,028 pounds is 16.7% to 39.2% MAC. The calculated centre of gravity on the load manifest form was 37.8% MAC.
Everything appeared to be within limits.
The ramp agent later estimated the weight of the two heavy bags to be between 70 and 80 pounds each. If you recall, the captain knew they had almost a hundred pounds wiggle room because of the child listed as an adult.
If the captain had added the extra weight of the two heavy bags, included the missing 110 pounds of fuel and then correctly recorded the 12-year-old passenger’s weight as 80 rather than as 175 pounds, then the total take-off weight would have been 17,093 pounds with a centre of gravity (CG) position of 38.8 percent MAC.
This is still within the envelope of the Beechcraft 1900D weight and centre of gravity envelope; these mathematical errors did not explain the accident.
In order to find out the true weight, investigators collected every piece of the aircraft and its contents. The luggage, carry-on and checked-in, the personal effects of the crew, the manuals and the logs were all dried out and weighed. The medical examiner collected the actual weights of the crew and passengers. The cargo (a tyre in the aft compartment) was weighed separately, as were the contents in the coat closet.
The Safety Board redid the load manifest form with these actual weights from the aircraft. They discovered that flight 5481 had a minimum gross take-off weight of 17,443 pounds and a centre of gravity position of 43.3 percent MAC, which was outside of the envelope.
Four of the checked-in bags weighed more than 50 pounds, twice the average weight of 25 pounds allotted. The heaviest bag weighed 69 pounds. The total baggage weight in the hold was underestimated by at least 124 pounds.
Then there was the estimated weight of the passengers. The average weight of 175 pounds per adult was commonly used at the time, but it was low. In all actuality, the average weight of the passengers on flight 5481 was 185 pounds.
Advisory Circular 120-27C Aircraft Weight and Balance Control, issued in November 1995, suggested an average weight for adults as 180 pounds in the spring and summer months and 185 pounds in the autumn and winter. However, they also warned that these were “standard, average weights for a conventional passenger group” and recommended that air carriers conduct a reliable survey to establish the correct average weights for their operations.
After the accident, the FAA requested all operators of 10 to 19 passenger-seat aircraft to conduct such a survey to validate the average weights that they were using. Operators either asked passengers their weight and then added ten pounds (for personal items), or they physically weighed the passenger on the spot. They also weighed all checked and carry-on baggage.
Operator A in the table was Air Midwest. The airline surveyed all of their flights over a 3-day period (540 flights) at 98 percent of their stations. They discovered that the average weight of their adults was 200 pounds, so with the winter average weight of 175 pounds, they were 25 pounds underweight per adult passenger.
The average checked bag was found to be 30 pounds, 5 pounds over the average used, but the bigger issue was that the weight of carry-on bags had increased dramatically, averaging 20 pounds per person. The 175 pounds average weight per passenger included personal effects such as carry-on luggage, so this additional weight was more than double what they were expecting.
Air Midwest have revised their policy to use the new average weights of 200 lbs per adult and 30 lbs for checked baggage. This change alone reduced the Beechcraft 1900D aircraft passenger capacity from 19 passengers to 17.
Having proved that the aircraft was overweight with the centre of gravity outside of the limits, investigators still could not explain the accident. This caused the nose to pitch up, without a question; however the captain responded quickly and confidently. Under the circumstances, she could and should have recovered control of the aircraft.
Next week, we’ll take a look at what actually went wrong with the aircraft.
This is a sad story and we will have to wait till next week when Sylvia promised to give her readers the final findings to explain why the crew lost control and failed to recover.
But it seems clear that the centre of gravity must have been out of limits. Was there some cargo or heavy luggage that was not, or not weighed properly put in the aft compartment? Were heavy passengers seated in the back? Last week I already commented in another blog here that passengers today are generally heavier than the outdated “standard” figures and Americans especially so. Then there is the seasonal variation: heavier clothes in winter. Weight and balance is a very serious matter. The Fokker F27s that I flew were converted from passenger- to cargo configuration. The interior had been stripped and a reinforced floor with cargo anchoring points replaced the lighter aluminium floor of the passenger version. The galley that had been installed in the rear had been removed and a large, electrically operated cargo door was built in the forward section, in front of the port propeller. And of course this also required a re-enforced door frame that could be sealed when the cabin was pressurised.
The result was that the aircraft required 500 kg. ballast in the tail if no cargo was carried. At least, during take-off the first thing that pilots would notice if no ballast were carried was that rotation would require a lot of back pressure on the elevator. The Beech 1900D obviously required the opposite, the elevator (I presume that this information was sourced from the flight data recorder) was 7 degrees nose-down and the aircraft rotated at 102 kts. Sylvia mentions that this type of aircraft requires a speed of 105 kts to safely lift-off. It is not unusual for small regional airliners to have a “one speed fits all”, rather than a calculated set of V-speeds but the aircraft was already a bit heavier than the loadsheet suggested, and it lifted off at 3 kts slower than the recommended safe speed, a speed that probably has been calculated for the maximum take-off weight, so with a heavy aircraft there was not a lot of leeway. The accident at that moment was probably already unavoidable.
If I go into the realm of pure speculation, forgive me, this may be total “bovine manure”, but I try anyway:
Sylvia already explained that ideally the centre of gravity should be at, or close to the wings’ MAC. There are certain limits by which this amount is allowed to vary.
The elevator is a movable horizontal control surface, attached to the horizontal tail plane. This assembly is in itself a small wing.
The lift generated by the wing depends on the speed of the aircraft and the angle of attack, an angle between the air meeting the wings. The design of an aircraft partly meets this requirement by fixing the wing itself under a certain angle to the fuselage, this is the angle of incidence.
A take-off flap setting will be unchanged until the aircraft is safely airborne, is climbing and has accelerated to the speed where they may be retracted. During take-off they do not move, so they are not part of this equation, But the elevators can be moved during any stage of flight. By moving the control column forward the angle of attack is increased, the tail is given extra lift to compensate for a tail-heavy load situation. But there are limits, the elevators not only control or compensate for a centre of gravity that may be a bit forward or aft of the MAC. The elevators are also needed to control the aircraft attitude during flight, to pitch up or down as the situation may require and also control the aircraft’s pitch during landing. A larger than normal elevator deflection also creates extra drag. A tail-heavy situation that requires a lot of forward force on the controls is a very dangerous one.
But, and here I start speculating:
The aircraft lifted off prematurely and with 7 degrees forward (nose down) elevator, yet, the aircraft assumed a very steep climb.
My guess is that when the aircraft went into an aerodynamic stall, the tailplane had already stalled first. This is not supposed to happen, but remember that the weight and balance of the aircraft was out of limits, probably by a substantial amount. At the same time the elevator was down, meaning that its angle of attack was high. It was unable to generate the lift – remember: not the lift of the wings – needed to bring the tail up (= nose down) and recover from the stall. The tailplane stalled first, the aircraft entered a “deep stall”. Even if there had been sufficient altitude to recover, the out of c of g situation prevented this; the tail (elevator) had to un-stall first before it would have any effect, the aircraft was uncontrollable and that at a very low altitude. Recovery sadly was not possible.
One last remark: Fuel is measured, not in gallons or litres, but in weight, usually in pounds..
As Sylvia explained (correctly of course), this is important for the weight and balance calculations, but there is another, equally important reason: Fuel – and especially jet fuel but it also is valid for AVGAS – has a specific caloric efficiency that depends on its weight, NOT the volume.
So if my private Wonderjet is refueled from a bowser that has been parked most of the day in the sunshine of a hot summer’s day in Palma de Mallorca, the fuel will have expanded – measurably so.
This is not hypothetical, it happened to me. When it came to refuel our Wonderjet (actually it was an Aerospatiale SN 701 “Corvette”),one day, we needed the maximum amount of fuel in order to fly home non-stop.
When the tanks were full (the aircraft had a pressure refueling system) we were several hundreds of pounds UNDER the normal maximum. The fuel had expanded, and its specific gravity was lower. We had received the full quantity in volume, but not in weight.
The energy that can be extracted from a pound of fuel remains the same, regardless of the volume. With full tanks, that day our range was reduced by several hundreds of nautical miles.
How did you measure the fuel shortage? Fuel-truck weight before/after? Temperature of the fuel as it went in? SG of a sample of the fuel?
We have scales that weigh fully laden long haul trucks, but we can’t be bothered to weigh passengers or their luggage. In ancient Rome the wealthy sometimes used lead cookware. Are we any smarter?
We have truck scales; they’re frequently unused.
Overloading a truck is easy; it’s a big box with a load capacity around 10 pounds per cubic foot. (Water is ~62 #/cf; that’s why carbonated drinks aren’t delivered in semis.) Not a good analogy to a seating compartment.
As Sylvia’s tables show, the issue started with an unrealistic average weight. Fixing that costs a lot less (in money, time, and customer difficulties) than instituting a universal weighing plan.
I wonder how accurate Rudy’s statement is that USians are heavier than other airplane passengers; I also wonder about regional variations. (Note that this airline’s passengers were heavier than any others — but we don’t know anything about the methodology beyond the fact that they checked (somehow) almost everyone’s weight over a short period.)
missed thought — I wonder how much the increase in hand-luggage weight comes from the airlines’ charging for checked bags? ISTM that more and more people are trying to carry on everything — although I don’t know how possible that is in this size aircraft. (The few aircraft this size I remember riding in had much less space for carry-ons than standard passenger airplanes.)
We don’t weigh because somebody decided the risk of using averages and getting it wrong was outweighed by the cost of installing scales, establishing procedures, and dealing with all the data appropriately.
This type of judgement has been a hallmark of civilization since the dawn of time. You can define power as who decides who assumes the most risk.
Mike, I guess that you will have to ask the airlines’ commercial departments that question. Of course, with the current trend to check in at home by computer, the options of determining the actual weight of the passengers are becoming even more limited. With the covid-19 restrictions severely reducing the numbers of travelers this would not be too much of a problem. If airports are full of people, all with their boarding pass pre-printed arriving at the gate, this is becoming a virtual impossibility. When I was flying with Ryanair (in the good old days before Michael O’Leary) we were well aware of the differences that could exist when determining weight using “standard weights”.
When checking the loadsheet, I always made a mental calculation by adding 10 kg per male, and 5 per female passenger on the paperwork and on cold days an extra 2 kg for extra heavy clothing.
I never came across a difference that was large enough to bring the aircraft over the take off limits, but we used the heavier weight figure to calculate our V-speeds and EPR. On one occasion the difference of that small extra weight just was enough to bring us in the sector where we had to use water injection.
This meant that we had to leave the cockpit, because the demineralised water, once in the reservoir, had to be used. We carried a drum and a pump in one of the forward belly holds. It took about 10 minutes to fill it. We had to wait for our departure slot time, so it did not cause any delay.
If it had been raining we added 1 kg per passenger to allow for the extra weight of the water that had been absorbed in clothing.
Again, these calculations were something that we (not every crew) did above and beyond the regular and official W&B and it was a mental exercise that we did if the aircraft was going to be heavy. It was important for Coventry, because the runway was very limiting for the BAC 1-11.
A member of the ground staff swore that when rotating, the nose of the aircraft was already over the grass at the end before the main wheels left the ground. Of course, even if this were to be true (I strongly doubt it), V-1 would have been reached well before V-r.
What was interesting though is that if our calculations were correct, we also would have burned 100 lbs extra fuel (on a 45 minute flight from Dublin to Luton). Fuel burn was a major factor. Most captains wanted 7.5 tons as standard on this leg. One of the training captains who checked me out never took more than 6500 kg. His argument, which he demonstrated to me, was that the extra fuel weight resulted in an increased fuel consumption, just to carry it.
It all goes to demonstrate how important it is to pay serious attention to the correct aircraft weight. Its dividends are not just enhanced safety, but also in saving money.
Thank you for another well-written accident report that ties in well with last week’s topic and made me curious enough to look up the accident report. ;-)
I wonder what exactly a “crash phone” does. Do all airports have one?
I looked up what the “mean aerodynamic cord” means; there’s a detailed technical writeup at https://code7700.com/aero_weight_and_balance.htm . The upshoot is that it might as well be a measurement in cm or inches behind some arbitrary “datum point” of the plane; I think they only use the percentage measure to escape ambiguity as to what the measurement is referring to (lots of lengths on an aircraft, but only one MAC) and to become metric/imperial-agnostic. It would really be useful if the center of lift was always at 50% MAC, but that can’t be the case because the lift of the wing (and where it is generated) changes with the speed of the aircraft.
The general idea is for the stabilizer in the tail to generate a downward moment that is balanced by an equal downward moment from the weight of the aircraft on the other side of the center of lift, which acts like a fulcrum on a see-saw. This arrangement causes the nose to tip down if the stabilizer ever stalls, which helps recover from the stall.
I know that the phrase “geographic center” has been copied all over the place, but I doubt geography plays a role in aircraft construction?
Another thing I looked up after reading the original accident report is what a “travel board” looks like, and how it is used; I bet I’d have learned that in next week’s blog post if I’d been more patient!
Here’s my primary reference: https://www.faa.gov/regulations_policies/handbooks_manuals/aviation/media/faa-h-8083-1.pdf
(it definitely says that “you can also think of it as the chord drawn through the geographic center of the plan area of the wing” but I just presumed that was meaningful to people who didn’t fail geometry.)
I struggle with some of this. My notes on MAC summarise my understanding as “The Mean Aerodynamic Chord is an imaginary line of an imaginary aerofoil”.
I might take another run at it but not this week.
Chip,
The fuel gauges of the SN 601 (not “701”, that was a typo) and most if not all aircraft get their information from capacitators. The gauges are calibrated in weight. The good thing is that the resistance of the fuel in the tanks changes with the density, so the indications are correct regardless.
As mentioned: on a hot day the tanks may be physically full by volume, but the less dense fuel has less total weight. A typical car fuel indicator works with a float. So it will indicate volume. An aircraft gauge gets its information from the standpipe capacitator, which is indicating the correct weight of the fuel on the dials in the cockpit.
Mendel,
You are getting beyond me now.
The optimum position of the C of G is around the MAC. The elevator provides the up- or downward force (lift) to compensate for limited deviations and for the control of the aircraft.
A “crash phone” is often a fixed line (red phone) in the control tower usually next to a button that sounds a loud horn or siren to put all rescue staff on immediate alert. The phone provides a direct connection with the fire chief or such other senior official who will answer it to get the information (s)he needs for an effective response.
PS:
Mendel, insofar as I am aware the elevator is not designed to impose a permanent upwards force = lifting the tail section for immediate stall recovery.
No, I disagree with that – maybe I misunderstood you.
BUT: it is supposed to remain effective well after the wings stall. In that case, it will of course still provide lift which, in turn, will help recovery from a stall.
The Beech 1900 that was involved in this tragic accident seems to have been grossly out of C of G limits. So far out, in fact, that the aircraft lifted off prematurely (at 102 kts iso 105), with a 7 degree down elevator deflection (“down deflection” meaning: increasing lift to keep the nose down !) and yet, it assumed a steep climb.
My guess – and it was no more than that – was that the aircraft’s c of g was so far out of kilter that the elevator also stalled, at or around the same time as the wings, maybe even before the wings.
In that HYPOTHETICAL case the elevator would not be able to control the pitch and assist stall recovery.
Geographical centre? No that makes no sense to me, unless it is refers to a theoretical datum point for the weight and balance calculations. For the Citation 500 series a point was chosen about a metre in front of the aircraft. That worked fine, and I suppose prevented mistakes due to “in front of” or “behind” the MAC. The original basic calculations were more complex, but Cessna did this for the owners anyway. These owners, not seldom with a PPL, had an easier job calculating the correct aircraft balance for take-off.
I think “geographical centre” is an autocorrected version of “geometric centre”–the midline found by calculating the wing’s shape/dimensions in planview.
Hmm: I quoted the FAA handbook above but I do not know enough geometry to know if geometric centre is the better phrase!
Google and Google books do not make much of a distinction between “geographic center” and “geometric center” in regards to airfoils. However, the Internet Archive (a collection of PDFs of full-text books and magazines, most of of print) definitely prefers the latter (4;1 ratio), and most works in which the former appear discuss both surveying AND aviation OR date from the 21st century, suggesting to me that one idiot got it wrong on the internet and many other idiots copied and pasted the first idiot’s error. i am a historian (of military aviation), and see this phenomenon with distressing regularity.
+ a quick survey of the Hathi Trust, another online archive of full-text PDFs, shows an even greater disparity (7:1), and offers much evidence to support my earlier remarks about surveying & aviation, the 21st century, and idiots.
I’ve been coming to the same conclusion so I’m glad to see your comment supporting this. I will come back to the subject in a new post (but first we need to finish Air Midwest 5481)
Ah Roger, that does make more sense now.
For the record: I think (hope) that it was clear that I was putting random thoughts that came in my head in my reactions here.
Those who (correctly) think that the tailplane (elevator) is not supposed to stall before the wings: that is absolutely true. The wing is attached to the fuselage at a positive angle, the angle of incidence*. If the aircraft moves straight through the air, this angle becomes the angle of attack, but by raising (or lowering) the nose, the angle of attack can vary.
The horizontal stabilizer, the tailplane, is attached with a neutral angle of incidence. This should ensure that the wings will stall before the elevators, meaning that the pilots will have pitch control and will be able to recover from the stall.
I was just trying to figure out for myself if a situation where the aircraft would be very much outside of W&B limits, this could be compromised to the point where the tail stalls first, or at the same time as the wings.
Normally, when lifting off, or bringing the nose up, the elevator would also be raised and the angle of attack reduced. But in the case of this accident, the aircraft lifted off with a downward elevator angle: a higher tailplane angle of attack than normal and still the pitch kept increasing. The crew would have lost “down” elevator authority just when they needed all that should have been available.
The aircraft would have virtually, literally, “fallen out of the sky” before somehow sufficient airspeed could be regained to recover.
Reading the sequence of events in this accident I have the feeling that this seems to have been the case.
* Aircraft designed specifically for aerobatics may have a zero wing angle of incidence. This improves the handling when flying inverted.
Sylvia,
It will be best if we leave the definition of MAC to you.
There are two others:
– Military Air Transport Command,
– Martin’s Air Charter. Because of the confusion with the US military, the company was later rebranded as “Martinair”. It is now the air cargo arm or KLM.
The easiest way that we, as trainees pilots were given as explanation was an imaginary line over the wing, from the root to the tip, where under normal flight conditions the wing provided the most of its lift.
But then, it was later explained that it was the line where the thickness was greatest. Both are more than likely inaccurate.